Habitat changes
As the transformation of sea-ice habitats progresses, especially those organisms that directly or indirectly depend on ice algae for food – like sea-ice amphipods or copepods, which are an important food source for the Arctic cod and Greenland cod (Boreogadus saida, Arctogadus glacialis) – will lose their habitat. The ice is melting earlier in the year and freezing later than before. These changes could have significant impacts on the lifecycles of those fauna that feed on ice algae – particularly those that have adapted to the ice habitat and aren’t suited to life in the water column (Lannuzel et al., 2020).
For example, some ice-associated amphipods and copepods have adapted their reproductive cycles to the formation of algae blooms. They reproduce when the blooms are at their peak, providing ample food. If the blooms formed earlier due to thin ice (and more available light), the effects could be disastrous, because the freshly hatched amphipods and copepods wouldn’t find enough food. As such, the populations of these species may decline considerably in the future. Further, if they serve as food for higher organisms like the Arctic cod and Greenland cod, the stocks of these fishes could also be in jeopardy (Lannuzel et al., 2020).
If the sea ice melts earlier and forms later, it will also mean that the ice algae season as a whole is shorter. Experts predict that, especially in the eastern Arctic, this aspect will mean a lack of food for ecologically important species like amphipods, copepods and the Arctic cod. Although increased primary production is expected for some regions of the Arctic, this mainly applies to the pelagic algae, not the ice algae. With the growing selection of pelagic algae, those consumers that feed on these algae or smaller zooplankters – including predatory amphipods like Themisto, which e.g. are now being found farther south in the Barents Sea; Arctic krill species; and jellyfish – are likely to penetrate deeper into the Arctic (Lannuzel et al., 2020).

Especially large zooplankters that are rich in valuable fatty acids feed on sea-ice algae, and are in turn important food sources for higher organisms like fishes. When it comes to the coastal shelf regions, with the loss of sea ice, experts expect to see free-swimming zooplankters replace the sea-ice zooplankton – e.g. smaller copepods, including Pseudocalanus and Metridia species. When the nutritious zooplankton on the sea ice disappear, those species that have specialised in this type of food will likely become correspondingly more seldom. In regions that are still dominated by the Arctic cod today, more sand lances, which are less nutritious by comparison, could migrate from the south. In the process, the loss of energy-rich food would progress from the Arctic cod to those animals that feed on it. A prolonged decline in the most important prey species like the Arctic cod will likely exacerbate the decline in ice-associated predators like ringed seals, whales and polar bears that has already been observed. As a result, these mammals could die out in large expanses of their distribution areas (Lannuzel et al., 2020).
Accordingly, experts now assume that the sum total of all these changes will most likely reduce the biomass in the Arctic – despite seasonally higher primary production in the ice-free waters. Changes in the biodiversity are also expected: typical Arctic species could disappear or withdraw to smaller refuges where better living conditions can still be found. At the same time, typical Atlantic or Pacific flora and fauna species are increasingly migrating to the Arctic from the south. As the Arctic gradually becomes ‘more Atlantic’ and ‘more Pacific’, its original biodiversity continues to decline (Lannuzel et al., 2020). The common higher animals that are likely to head farther north include baleen whales and various seabirds, which normally avoid the ice (IPCC, 2019).
Researchers have also recently observed a very different type of change in ringed seals in Svalbard. Since many parts of the coast are ice-free during the summer months, the seals now gather in large numbers on the shores of the islands, not on the ice – which automatically puts them at a greater risk of encounters with predators like polar bears. This could be an example of how fauna are now adapting to the extreme changes in their habitats, produced by the loss of sea ice (IPCC, 2019).
In the Antarctic, too, the loss or thinning of the sea ice will significantly change the microalgae communities. Organisms that depend on ice algae will be left empty-handed, while algae species that live in the open water and/or at higher temperatures will thrive. But the loss of sea ice also has direct impacts – e.g. for seals, which use the ice as their hunting grounds, or as a refuge for bearing their young. In the Antarctic Peninsula region, experts have long since observed major changes in the composition of the algal biomass: from 1998 to 2006 the volume of algae living in the open water increased in the south. This can be attributed to sea-ice retreat, more intensive mixing of the surface water, and with it, more available nutrients and freshening of the seawater due to input from glacier water. Here, too, the large diatoms are disappearing, while smaller algae species, especially the so-called cryptophytes, are on the rise. This shift in the algal composition, which continues to spread farther south, has most likely already contributed to changes in the food webs off the Antarctic Peninsula, where e.g. Antarctic silverfish (Pleuragramma antarctica) stocks have diminished (Massom et al., 2010). The fish spawn on the sea ice, where their larvae subsequently grow. As such, they are hard hit by the loss of sea ice off the Peninsula. The Antarctic silverfish is the most common fish in the waters off the Antarctic coast and the preferred food for the Adélie penguin and many other predators like the Weddell seal. The declining fish stocks are likely to become increasingly problematic for these species (IPCC, 2019).
On the other hand, there are also winners. The change from large diatoms that live on the sea ice to smaller algae in the open water would appear to be a boon for salps, a form of transparent tunicate. Because they filter large amounts of algae from the water, they are considered competitors for fish. The problem: salps are far less nutritious than fish, which means they can’t offer higher animals as much energy. As such, they’re most likely no substitute for the fish stocks now in decline (Massom et al., 2010).
One species particularly affected by the changes in the Antarctic is the Adélie penguin, whose population on the Antarctic Peninsula has shrunk by 65 percent over the past 25 years. At the same time, the stocks of gentoo penguins and chinstrap penguins have grown. Both species feel less at home in central Antarctica and more in the sub-Antarctic zone, are adapted to warmer conditions and need no sea ice. Exactly why the number of Adélie penguins continues to decline remains unclear; there appear to be a number of reasons. Firstly, more intensive storms and snowfall during the breeding season could be a factor. Since Adélie penguins begin breeding in spring, their young may be impacted more by the harsh conditions. Gentoo and chinstrap penguins breed later, when the weather is more clement. Another reason could be a lack of food in spring, when the young are nurtured. If so, this would be due to the dwindling stocks of Antarctic silverfish and Antarctic krill (Massom et al., 2010).
In this regard, it should be kept in mind that penguin stocks can also develop quite differently in different parts of the Antarctic. For example, on the outliers of the Antarctic Peninsula near Signy Island, both Adélie penguin and chinstrap penguin populations are in decline, while gentoo populations are growing (Massom et al., 2010). This trend is attributed to periodic warm spells, which reduce the amount of sea ice, and with it, the available food. Here the relations are still poorly understood. It also remains to be seen whether the penguin species, especially the Adélie penguin, can adapt to these new conditions. Some experts have suggested that changes in the Adélie penguin populations could be the result of natural environmental fluctuations from year to year, i.e., that there are simply better and worse years in terms of their prey and reproductive success – with no connection to climate change (IPCC, 2019).
In the Ross Sea, on the other side of the Antarctic continent, the situation is completely different. Here the Adélie penguin populations have been growing for years, presumably due to the good sea-ice conditions. In addition, local winds often produce polynyas, patches of open water in the ice where the penguins can hunt. In East Antarctica, the number of Adélie penguins is also on the rise (Massom et al., 2010).

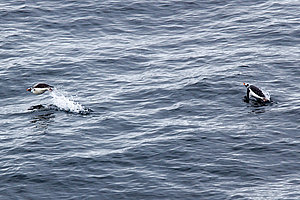

When it comes to the emperor penguin populations, experts are currently reluctant to make any estimates. Recent findings indicate that the latest estimates should be taken with a grain of salt. It is believed that a number of breeding colonies have yet to be discovered and/or verified. Trend-based estimates and conclusions drawn on the basis of individual col-onies are viewed critically (IPCC, 2019).
Further, it remains difficult to estimate the population development for those seals that have close ties to the sea ice – including the Ross seal, crabeater seal, leopard seal and Weddell seal. First of all, distinguishing between the influence of anthropogenic climate change and natural, recurring climatic fluctuations is difficult. In addition, there is no uniform global estimate for the number of Antarctic pack-ice seal species. As such, there is no reference data that would allow us to compare changes in seal populations due to climate change; currently, only regional estimates are available (IPCC, 2019).
The situation is similar when it comes to estimating the status quo for whales. Like seals, whales live for many years. In addition, they travel considerable distances, which makes it difficult to recognise changes in their populations, let alone determine whether or not they resulted from climate change. Given the lack of data, it is virtually impossible to say at this point how the latest changes in the sea ice have affected ice-associated species like the minke whale, blue whale and killer whale (Massom et al., 2010). Birds living near the sea ice will most likely also be affected; the populations of Antarctic petrels and snow petrels could decline (IPCC 2019).
Krill decline off the Antarctic Peninsula

Euphasia superba is by far the most dominant krill species in the Southern Ocean. Given its distribution throughout the Antarctic, estimates of the overall biomass are difficult, currently ranging from 122 to 215 million metric tons. Older estimates are as high as 379 million tons. Krill feed on microalgae and are in turn an important food source for penguins, seals and whales. Experts have only recently come to recognise the krill’s tremendous importance for Antarctic nutrient cycles from the water’s surface to the seafloor: the krill is the central species in the Antarctic, connecting all levels of the food web (Cavan et al., 2019).
The largest krill populations can be found in the Atlantic sector of the Southern Ocean, reaching to the Antarctic Peninsula. Yet this is also the region of the Antarctic in which climate change can most clearly be seen; the sea ice, shelf ice and glaciers are melting faster than elsewhere (IPCC, 2019). Although the ice-free waters are boosting primary production, which especially those marine organisms that feed on small planktic algae species are benefiting from, at the same time, less sea ice means less ice algae. This is worrisome, since for the krill, successful reproduction directly depends on the sea ice; the ideal conditions are harsh winters with extensive sea ice under which its larvae overwinter, followed by large ice-algae blooms dominated by energy-rich diatoms in spring. Under these conditions, by summer there can be a strong new generation of krill. Accordingly, the steady decline in sea ice with a trend toward smaller phytoplankton species is producing declining krill populations. How these populations will change in the Antarctic as a whole, and particularly off the Antarctic Peninsula, in the years to come is the subject of an ongoing academic discourse. One aspect that makes assessing the situation more difficult: natural climate variations at intervals ranging from several years to several decades, which overlap with the effects of anthropogenic climate change. Not all scientific studies clearly attribute the development of krill populations to anthropogenic climate change. But for the southwest Atlantic, there is compelling evidence that the populations are declining in step with the ice – a disastrous trend, since these waters are home to essential areas where krill spawn and where their young mature. When winter conditions are poor, the krill appear to retreat farther south, to the remaining sea-ice regions. If the krill biomass truly does drop significantly in the years to come, it will also affect the penguin, seal and whale populations (Lannuzel et al., 2020).
By consuming enormous amounts of algae, the large krill populations make a major contribution to nutrient recycling in the ocean. In this regard, their up to one-centimetre-long faecal pellets, which transport nutrients and carbon from the surface water to the seafloor, are particularly important. Since the pellets are hard and dense, they sink rapidly, giving bacteria little chance to break them down along the way. Further, since krill and krill larvae engage in vertical migration over the course of the day (diurnal migration), many pellets are released at lower depths, accelerating their transport to the seafloor. But their faeces aren’t the only way in which krill contribute to nutrient cycles; their exoskeletons, which they shed (moult) roughly every two weeks, also transport considerable amounts of nutrients to the deep. Moreover, krill recycle nutrients near the surface: by eating algae, they release nitrogen compounds and other substances, making them available to the plants once again (Cavan et al., 2019). All in all, krill represent a central element in Antarctic nutrient cycles. Experts fear that these cycles could experience major disruptions if the krill populations suffer a major decline due to climate change and dwindling sea ice.
Cavan, E.L., A. Belcher, A. & A. Atkinson et al. (2019): The importance of Antarctic krill in biogeochemical cycles. Nat Commun 10, 4742. doi.org/10.1038/s41467-019-12668-7
Grosfeld, K. , R. Treffeisen & S. Löschke (2020): DriftStories aus der zentralen Arktis - Ein Jahr, eine Scholle - Meereisforschung extrem / K. Grosfeld , R. Treffeisen and S. Löschke (editors), Bremerhaven, REKLIM - Helmholtz-Verbund Regionale Klimaänderungen und Mensch, 106 p., ISBN: 978-3-9822680-0-2.
Henley, S.F. et al. (2020): Changing Biogeochemistry of the Southern Ocean and Its Ecosys-tem Implications. Frontiers in Marine Science, 7, 581.
IPCC, 2019: IPCC Special Report on the Ocean and Cryosphere in a Changing Climate [H.-O. Pörtner, D.C. Roberts, V. Masson-Delmotte, P. Zhai, M. Tignor, E. Poloczanska, K. Mintenbeck, A. Alegría, M. Nicolai, A. Okem, J. Petzold, B. Rama, N.M. Weyer (eds)].
Lannuzel, D., L. Tedesco & M. van Leeuwe et al. (2020): The future of Arctic sea-ice bio-geochemistry and ice-associated ecosystems. Nat. Clim. Chang. 10, 983–992. doi.org/10.1038/s41558-020-00940-4
Maribus gGmbH (Ed.) (2019): Arktis und Antarktis – extrem, klimarelevant, gefährdet. In: World Ocean Review, Band 6.
Massom R. A., et al. (2010): Antarctic sea ice change and variability e Physical and ecologi-cal implications. Polar science 4, 149-186.